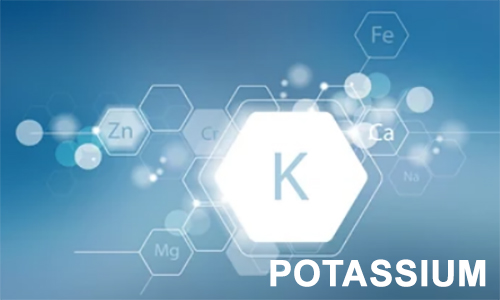
Please consult with your health-care provider before making any dietary or other changes based on any of the information provided here.
If you live in Quebec and do not have a family doctor, you can [ sign up here ].
Anatomy and Physiology
Anatomy and Physiology is a dynamic textbook for the two-semester human anatomy and physiology course for life science and allied health majors. The book is organized by body system and covers standard scope and sequence requirements.
More InfoHuman Nutrition
This textbook serves as an introduction to nutrition. The book covers basic concepts in human nutrition, key information about essential nutrients, basic nutritional assessment, and nutrition across the lifespan.
More InfoPrinciples of Nutrition
A great textbook to get started on a journey into the world of health and nutrition. The first focus will be to demonstrate that nutritional science is an evolving field of study, continually being updated and supported by research, studies, and trials.
More InfoMicrobiology
Microbiology covers the scope and sequence requirements for a single-semester microbiology course for non-majors. The book presents the core concepts of microbiology with a focus on applications for careers in allied health. The pedagogical features of the text make the material interesting and accessible while maintaining the career-application focus and scientific rigor inherent in the subject matter.
More InfoChemistry
Chemistry 2e is designed to meet the scope and sequence requirements of the two-semester general chemistry course. The textbook provides an important opportunity for students to learn the core concepts of chemistry and understand how those concepts apply to their lives and the world around them. The book also includes a number of innovative features, including interactive exercises and real-world applications.
More InfoBiology
Biology 2e is designed to cover the scope and sequence requirements of a typical two-semester biology course for science majors. The text provides comprehensive coverage of foundational research and core biology concepts through an evolutionary lens. Biology includes rich features that engage students in scientific inquiry, highlight careers in the biological sciences, and offer everyday applications.
More InfoHypokalemia | Normal Range of 3.5 – 5.1 mmol / L | Hyperkalemia
Potassium
Potassium is the most abundant positively charged ion inside of cells. Ninety percent of potassium exists in intracellular fluid, with about 10 percent in extracellular fluid, and only 1 percent in blood plasma. As with sodium, potassium levels in the blood are strictly regulated. The hormone aldosterone is what primarily controls potassium levels, but other hormones (such as insulin) also play a role.
When potassium levels in the blood increase, the adrenal glands release aldosterone. The aldosterone acts on the collecting ducts of kidneys, where it stimulates an increase in the number of sodium-potassium pumps. Sodium is then reabsorbed and more potassium is excreted. Because potassium is required for maintaining sodium levels, and hence fluid balance, about 200 milligrams of potassium are lost from the body every day.
Potassium and the Nervous System
Nerve impulse involves not only sodium, but also potassium. A nerve impulse moves along a nerve via the movement of sodium ions into the cell. To end the impulse, potassium ions rush out of the nerve cell, thereby decreasing the positive charge inside the nerve cell. This diminishes the stimulus.
To restore the original concentrations of ions between the intracellular and extracellular fluid, the sodium-potassium pump transfers sodium ions out in exchange for potassium ions in. On completion of the restored ion concentrations, a nerve cell is now ready to receive the next impulse.
The Central Nervous System
The central nervous system (CNS) is made up of the brain and spinal cord and is covered with three layers of protective coverings called meninges.
(“meninges” is derived from the Greek and means “membranes”)
The outermost layer is the dura mater, the middle layer is the web-like arachnoid mater, and the inner layer is the pia mater, which directly contacts and covers the brain and spinal cord.
The space between the arachnoid and pia maters is filled with cerebrospinal fluid (CSF). The brain floats in CSF, which acts as a cushion and shock absorber.
The cerebral cortex is covered by three layers of meninges: the dura, arachnoid, and pia maters.
(credit: modification of work by Gray’s Anatomy)
The nervous system of the common laboratory fly, Drosophila melanogaster, contains around 100,000 neurons, the same number as a lobster. This number compares to 75 million in the mouse and 300 million in the octopus. A human brain contains around 86 billion neurons. Despite these very different numbers, the nervous systems of these animals control many of the same behaviors—from basic reflexes to more complicated behaviors like finding food and courting mates. The ability of neurons to communicate with each other as well as with other types of cells underlies all of these behaviors.
Most neurons share the same cellular components. But neurons are also highly specialized—different types of neurons have different sizes and shapes that relate to their functional roles.Like other cells, each neuron has a cell body (or soma) that contains a nucleus, smooth and rough endoplasmic reticulum, Golgi apparatus, mitochondria, and other cellular components. Neurons also contain unique structures for receiving and sending the electrical signals that make communication between neurons possible. The actions of this pump are costly: one molecule of ATP is used up for each turn. Up to 50 percent of a neuron’s ATP is used in maintaining its membrane resting potential.
Dendrites are tree-like structures that extend away from the cell body to receive messages from other neurons at specialized junctions called synapses. Although some neurons do not have any dendrites, most have one or many dendrites. The bilayer lipid membrane that surrounds a neuron is impermeable to ions. To enter or exit the neuron, ions must pass through ion channels that span the membrane. Some ion channels need to be activated to open and allow ions to pass into or out of the cellThese ion channels are sensitive to the environment and can change their shape accordingly.
Ion channels that change their structure in response to voltage changes are called voltage-gated ion channels. The difference in total charge between the inside and outside of the cell is called the membrane potential. A neuron at rest is negatively charged: the inside of a cell is approximately 70 millivolts more negative than the outside (–70 mV). This voltage is called the resting membrane potential; it is caused by differences in the concentrations of ions inside and outside the cell and the selective permeability created by ion channels.Sodium-potassium pumps in the membrane produce the different ion concentrations inside and outside of the cell by bringing in two K+ ions and removing three Na+ ions.
Potassium ions (K+), which are higher inside the cell, move fairly freely out of the neuron through potassium channels; this loss of positive charge produces a net negative charge inside the cell. Sodium ions (Na+), which are low inside, have a driving force to enter but move less freely. Their channels are voltage dependent and will open when a slight change in the membrane potential triggers them. A neuron can receive input from other neurons and, if this input is strong enough, send the signal to downstream neurons.
Transmission of a signal between neurons is generally carried by a chemical, called a neurotransmitter, which diffuses from the axon of one neuron to the dendrite of a second neuron. When neurotransmitter molecules bind to receptors located on a neuron’s dendrites, the neurotransmitter opens ion channels in the dendrite’s plasma membrane. This opening allows sodium ions to enter the neuron and results in depolarization of the membrane—a decrease in the voltage across the neuron membrane. Once a signal is received by the dendrite, it then travels passively to the cell body.
A large enough signal from neurotransmitters will reach the axon. If it is strong enough (that is, if the threshold of excitation, a depolarization to around –60mV is reached), then depolarization creates a positive feedback loop: as more Na+ ions enter the cell, the axon becomes further depolarized, opening even more sodium channels at further distances from the cell body. This will cause voltage dependent Na+ channels further down the axon to open and more positive ions to enter the cell. In the axon, this “signal” will become a self-propagating brief reversal of the resting membrane potential called an action potential.
An action potential is an all-or-nothing event; it either happens or it does not. The threshold of excitation must be reached for the neuron to “fire” an action potential. As sodium ions rush into the cell, depolarization actually reverses the charge across the membrane form -70mv to +30mV. This change in the membrane potential causes voltage-gated K+ channels to open, and K+ begins to leave the cell, repolarizing it. At the same time, Na+ channels inactivate so no more Na+ enters the cell. K+ ions continue to leave the cell and the membrane potential returns to the resting potential.
Neurons contain organelles common to other cells, such as a nucleus and mitochondria. They also have more specialized structures, including dendrites and axons.
At the resting potential, the K+ channels close and Na+ channels reset. The depolarization of the membrane proceeds in a wave down the length of the axon.It travels in only one direction because the sodium channels have been inactivated and unavailable until the membrane potential is near the resting potential again; at this point they are reset to closed and can be opened again. An axon is a tube-like structure that propagates the signal from the cell body to specialized endings called axon terminals. These terminals in turn then synapse with other neurons, muscle, or target organs.
When the action potential reaches the axon terminal, this causes the release of neurotransmitter onto the dendrite of another neuron.Neurotransmitters released at axon terminals allow signals to be communicated to these other cells, and the process begins again. Neurons usually have one or two axons, but some neurons do not contain any axons.Some axons are covered with a special structure called a myelin sheath, which acts as an insulator to keep the electrical signal from dissipating as it travels down the axon. This insulation is important, as the axon from a human motor neuron can be as long as a meter (3.2 ft)—from the base of the spine to the toes. The myelin sheath is produced by glial cells.
Along the axon there are periodic gaps in the myelin sheath. These gaps are called nodes of Ranvier and are sites where the signal is “recharged” as it travels along the axon. It is important to note that a single neuron does not act alone—neuronal communication depends on the connections that neurons make with one another (as well as with other cells, like muscle cells). Dendrites from a single neuron may receive synaptic contact from many other neurons. For example, dendrites from a Purkinje cell in the cerebellum are thought to receive contact from as many as 200,000 other neurons.
Potassium and Aldosterone Feedback
Sodium is reabsorbed from the renal filtrate, and potassium is excreted into the filtrate in the renal collecting tubule. Principally two hormones—aldosterone and angiotensin II, govern the control of this exchange.
Angiotensin II : Causes vasoconstriction and an increase in systemic blood pressure. This action increases the glomerular filtration rate, resulting in more material filtered out of the glomerular capillaries and into Bowman’s capsule. Angiotensin II also signals an increase in the release of aldosterone from the adrenal cortex.
In the distal convoluted tubules and collecting ducts of the kidneys, aldosterone stimulates the synthesis and activation of the sodium-potassium pump.
Sodium passes from the filtrate, into and through the cells of the tubules and ducts, into the ECF and then into capillaries. Water follows the sodium due to osmosis. Thus, aldosterone causes an increase in blood sodium levels and blood volume.
Aldosterone’s effect on potassium is the reverse of that of sodium; under its influence, excess potassium is pumped into the renal filtrate for excretion from the body.
The Renin-Angiotensin System Angiotensin II stimulates the release of aldosterone from the adrenal cortex.
Aldosterone : Recall that aldosterone increases the excretion of potassium and the reabsorption of sodium in the distal tubule. Aldosterone is released if blood levels of potassium increase, if blood levels of sodium severely decrease, or if blood pressure decreases. Its net effect is to conserve and increase water levels in the plasma by reducing the excretion of sodium, and thus water, from the kidneys. In a negative feedback loop, increased osmolality of the extra cellular fluid (which follows aldosterone-stimulated sodium absorption) inhibits the release of the hormone.
The Aldosterone Feedback Loop Aldosterone, which is released by the adrenal gland, facilitates reabsorption of Na+ and thus the reabsorption of water.
Potassium acts as a buffer in blood, playing a role in acid-base balance.
Next we present some reference values for blood plasma, cerebrospinal fluid (CSF), and urine for various ions. In a clinical setting, sodium, potassium, and chloride are typically analyzed in a routine urine sample. In contrast, calcium and phosphate analysis requires a collection of urine across a 24-hour period, because the output of these ions can vary considerably over the course of a day. Urine values reflect the rates of excretion of these ions. Bicarbonate is the one ion that is not normally excreted in urine; instead, it is conserved by the kidneys for use in the body’s buffering systems.
Dietary Reference Intakes of Potassium
The IOM based their AIs for potassium on the levels associated with a decrease in blood pressure, a reduction in salt sensitivity, and a minimal risk of kidney stones. For adult male and females above the age of nineteen, the adequate intake for potassium is 4,700 grams per day. The AIs for other age groups are listed in Table 3.8 “Adequate Intakes for Potassium
Age Group | mg/day |
Infants (0–6 months) | 400 |
Infants (6–12 months) | 700 |
Children (1–3 years) | 3,000 |
Children (4–8 years) | 3,800 |
Children (9–13 years) | 4,500 |
Adolescents (14–18 years) | 4,700 |
Adults (> 19 years) | 4,700 |
Electrolyte and Ion Reference Values
Remember that references values vary by age, gender, fitness, physical and when individuals suffer from medical conditions. Values further vary based on the laboratory or country, as people near the equator (hot climate) may have different needs from individuals in colder climates.
Name | Chemical symbol | Plasma | Urine |
Sodium | Na+ | 136.00–146.00 (mM) | 40.00–220.00 (mM) |
Potassium | K+ | 3.50–5.00 (mM) | 25.00–125.00 (mM) |
Chloride | Cl– | 98.00–107.00 (mM) | 110.00–250.00 (mM) |
Bicarbonate | HCO3– | 22.00–29.00 (mM) | —— |
Calcium | Ca++ | 2.15–2.55 (mmol/day) | Up to 7.49 (mmol/day) |
Phosphate | HPO42 – | 0.81–1.45 (mmol/day) | 12.90–42.00 (mmol/day) |
Institute of Medicine.
http://www.iom.edu/
Accessed September 22, 2017.
Bio availability of Potassium
Greater than 90 percent of dietary potassium is absorbed in the small intestine via passive diffusion and used to maintain its normal intracellular and extracellular concentration. Although highly bioavailable, potassium is a very soluble mineral and easily lost during cooking and processing of foods.
Fresh and frozen foods are better sources of potassium than canned. Potassium excretion via the kidney reflects to changes in dietary intakes, and is higher in increases healthy people post prandial, unless there is some depletion. Nonetheless, the kidneys are equipped to adapt to variable potassium intakes in healthy individuals
Figure: Each part of the nephron performs a different function in filtering waste and maintaining homeostatic balance.
(1) The glomerulus forces small solutes out of the blood by pressure.
(2) The proximal convoluted tubule reabsorbs ions, water, and nutrients from the filtrate into the interstitial fluid, and actively transports toxins and drugs from the interstitial fluid into the filtrate. The proximal convoluted tubule also adjusts blood pH by selectively secreting ammonia (NH3) into the filtrate, where it reacts with H+ to form NH4 . The more acidic the filtrate, the more ammonia is secreted.
(3) The descending loop of Henle is lined with cells containing aquaporins that allow water to pass from the filtrate into the interstitial fluid.
(4) In the thin part of the ascending loop of Henle, Na+ and Cl- ions diffuse into the interstitial fluid. In the thick part, these same ions are actively transported into the interstitial fluid. Because salt but not water is lost, the filtrate becomes more dilute as it travels up the limb.
(5) In the distal convoluted tubule, K+ and H+ ions are selectively secreted into the filtrate, while Na+, Cl-, and HCO – ions are reabsorbed to maintain pH and electrolyte balance in the blood.
(6) The collecting duct reabsorbs solutes and water from the filtrate, forming dilute urine.
(credit: modification of work by NIDDK
Kidney Function and Physiology
Kidneys filter blood in a three-step process. First, the nephrons filter blood that runs through the capillary network in the glomerulus. Almost all solutes, except for proteins, are filtered out into the glomerulus by a process called glomerular filtration. Second, the filtrate is collected in the renal tubules. Most of the solutes get reabsorbed in the PCT by a process called tubular reabsorption. In the loop of Henle, the filtrate continues to exchange solutes and water with the renal medulla and the peritubular capillary network. Water is also reabsorbed during this step. Then, additional solutes and wastes are secreted into the kidney tubules during tubular secretion, which is, in essence, the opposite process to tubular reabsorption. The collecting ducts collect filtrate coming from the nephrons and fuse in the medullary papillae. From here, the papillae deliver the filtrate,
now called urine, into the minor calyces that eventually connect to the ureters through the renal pelvis. This entire process is illustrated in the Figure.
Glomerular Filtration
Glomerular filtration filters out most of the solutes due to high blood pressure and specialized membranes in the afferent arteriole. The blood pressure in the glomerulus is maintained independent of factors that affect systemic blood pressure. The “leaky” connections between the endothelial cells of the glomerular capillary network allow solutes to pass through easily.
All solutes in the glomerular capillaries, except for macromolecules like proteins, pass through by passive diffusion. There is no energy requirement at this stage of the filtration process. Glomerular filtration rate (GFR) is the volume of glomerular filtrate formed per minute by the kidneys. GFR is regulated by multiple mechanisms and is an important indicator of kidney function.
Nutritional Status of Potassium
Normal serum concentrations of potassium range from about 3.5 to 5.0 mmol/L. As most potassium is located inside cells, assessment of the status is difficult through cells. Therefore the testing is carried out through the blood, which can provide some indication of potassium status, however the correlation reflects poorly with tissue potassium stores. Other methods to measure potassium status can be used including, measuring net potassium retention and loss; measuring the total amount of potassium or the total amount of exchangeable potassium in the body; and conducting tissue analyses through muscle biopsies.
Potassium Deficiency
Insufficient potassium levels in the body (hypokalemia) can be caused by a low dietary intake of potassium or by high sodium intakes, but more commonly it results from medications that increase water excretion, mainly diuretics. The signs and symptoms of hypokalemia are related to the functions of potassium in nerve cells and consequently skeletal and smooth muscle contraction, which include muscle weakness and cramps, respiratory distress, and constipation.
Severe potassium depletion (serum potassium level less than about 3.5 mmol/L) can cause the heart to have abnormal contractions and can even be fatal. It commonly affects hospitalized patients, usually because of the use of diuretics and other medications and rare amongst people with normal renal function. Moderate to severe hypokalemia (serum potassium level less than about 2.5 mmol/L) can cause polyuria and encephalopathy in patients with renal disease; glucose intolerance; muscular paralysis; poor respiration; and cardiac arrhythmias, especially in individuals with underlying heart disease
Hypokalemia can also be caused by a condition called refeeding syndrome, which is defined as the metabolic response to initial refeeding after a period of starvation. This is due to potassium’s movement into cells post starvation. In these cases, feeding is gradually introduced to control potassium levels. Magnesium depletion can contribute to hypokalemia by increasing urinary potassium losses. Magnesium depletion is also often seen in refeeding patients. Unmanaged refeeding syndrome could result in cardiac issues. hypomagnesemia and hypokalemia, both should be treated concurrently as indicated clinically.
Dietary Sources Of Potassium
Potassium is abundant in a range of plant and animal foods and in beverages. Fruits and vegetables are rich sources of potassium, including legumes and potatoes.
Meats, poultry, fish, milk, yogurt, and nuts also contain potassium. In regards to complex carbohydrates, whole-wheat flour and brown rice produce contain higher quantities of potassium compared to refined carbohydrates
Potassium Supplementation
Potassium supplementation comes in various forms including potassium chloride, potassium citrate, phosphate, aspartate, bicarbonate, and gluconate.
The Supplement Facts panel on a dietary upplement label declares the amount of elemental potassium in the product, not the weight of the entire potassium-containing compound.
Some dietary supplements contain potassium iodide in microgram amounts, but this ingredient serves as a form of the mineral iodine, not potassium.
Some studies indicate potassium gluconate in supplements has a similar absorption rate to potassium in potatoe.
Other research concludes that liquid forms of potassium chloride, (which is normally used to treat hypokalemia clinically) is absorbed faster that a coated tablet form of potassium chloride.
Solutions of Solids in Liquids
The dependence of solubility on temperature for a number of solids in water is shown by the solubility curves in the figure below.
Reviewing these data indicates a general trend of increasing solubility with temperature, although there are exceptions, as illustrated by the ionic compound cerium sulfate.
Figure : Graph shows how the solubility of several solids changes with temperature.
Several different units are commonly used to express the concentrations of solution
components, each providing certain benefits for use in different applications.
For example, molarity (M) is a convenient unit for use in stoichiometric calculations, since it is defined in terms of the molar amounts of solute species:
M = mol solute L solution
Because solution volumes vary with temperature, molar concentrations will likewise vary. When expressed as molarity, the concentration of a solution with identical numbers of solute and solvent species will be different at different temperatures, due to the contraction/expansion of the solution.
More appropriate for calculations involving many colligative properties are mole-based concentration units whose values are not dependent on temperature. Two such units are mole fraction and molality.
The mole fraction, X, of a component is the ratio of its molar amount to the total number of moles of all solution components: In table salt (NaCl), the Cl accounts for 60% of any given number of moles.
total mol
By this definition, the sum of mole fractions for all solution components (the solvent and all solutes) is equal to one. Molality is a concentration unit defined as the ratio of the numbers of moles of solute to the mass of the solvent in kilograms.
Magnesium enhances the absorption of potassium. Therefore, consuming potassium with magnesium rich foods, can optimise potassium absorption:
Magnesium rich foods include:
- Green leafy vegetables (e.g. spinach and kale)
- Fruit (figs, avocado, banana and raspberries)
- Nuts and seeds
- Legumes (black beans, chickpeas and kidney beans)
- Vegetables (peas, broccoli, cabbage, green beans, artichokes, asparagus, brussels
sprouts) - Seafood (salmon, mackerel, tuna)
- Whole grains (brown rice and oats)
- Raw cacao
- Dark Chocolate
- Tofu
- Baked beans
- Chlorella powder
A reduction in sodium and increase in potassium will help with the maintenance of potassium levels. Consuming sodium in excess cause an imbalance in your potassium levels. This is because the two minerals work closely in the maintenance of fluid balance. High intakes of sodium will eliminate potassium via urination, decreasing potassium concentrations. It is also important to note that a potassium rich diet can decrease sodium levels and help management hypertension.
Potassium toxicity
Hyperkalemia, an elevated potassium blood level, also can impair the function of skeletal muscles, the nervous system, and the heart.
Hyperkalemia can result from increased dietary intake of potassium. In such a situation, potassium from the blood ends up in the ECF in abnormally high concentrations.
This can result in a partial depolarization (excitation) of the plasma membrane of skeletal muscle fibers, neurons, and cardiac cells of the heart, and can also lead to an inability of cells to repolarize.
For the heart, this means that it won’t relax after a contraction, and will effectively “seize” and stop pumping blood, which is fatal within minutes.
Because of such effects on the nervous system, a person with hyperkalemia may also exhibit mental confusion, numbness, and weakened respiratory muscles.
Low-potassium fruits
Treatment is dependent on the severity and the root cause of hyperkalemia.However, it most likely there will be a cessation of all offending medicines immediately and the patient is placed. The use of sodium to modulate high levels of potassium remains unclear. Furthermore sodium bicarbonate has been shown to be effective only in patients with metabolic acidosis and not hyperkalemia alone.
On a low potassium diet. Low potassium foods include:
|
|
|
Direct Foods that high in potassium
- Potatoe
- Tomatoe
- Banana
- White beans/lentils/pulses
- Plain yogurt
Direct -Foods low in potassium
- Apple sauce
- Watermelon
- Onion
- Raddish
- Peas
Absorption – If levels are MILDLY low
- Seafood (salmon, mackerel, tuna)
- Whole grains (brown rice and oats)
- Raw cacao
- Dark Chocolate
- Nuts/seeds
Absorption – If levels MILDLY high
No conclusive research to suggest
certain foods and the use of sodium
to modulate high levels of potassium
remains unclear ponents.
Signaling Molecules and Cellular Receptors
There are two kinds of communication in the world of living cells. Communication between cells is called intercellular signaling, and communication within a cell is called intracellular signaling. Chemical signals are released by signaling cells in the form of small molecules that interact with proteins called receptors.
Cell-surface receptors are involved in most of the signaling in multicellular organisms. There are three general categories of cellsurface receptors: ion channel-linked receptors, G-protein- inked receptors, and enzyme-linked receptors.
Ion channel-linked receptors bind a ligand and open a channel through the membrane that allows specific ions to pass through. To form a channel, this type of cell-surface receptor has an extensive membrane-spanning region. In order to interact with the double layer of phospholipid fatty acid tails that form the center of the plasma membrane, many of the amino acids in the membrane-spanning region are hydrophobic in nature.
Conversely, the amino acids that line the inside of the channel are hydrophilic to allow for the passage of water or ions. When a ligand binds to the extracellular region of the channel, there is a conformational change in the protein’s structure that allows ions such as sodium, calcium, magnesium, and hydrogen to pass through.
Signaling Molecules
Produced by signaling cells and the subsequent binding to receptors in target cells, ligands act as chemical signals that travel to the target cells to coordinate responses. The types of molecules that serve as ligands are incredibly varied and range from small proteins to small ions like calcium (Ca2+).
Figure: Gated ion channels form a pore through the plasma membrane that opens when the signaling molecule binds. The open pore then allows ions to flow into or out of the cell.